Alumnus on Columbia University ‘Twistoptics’ Research Team
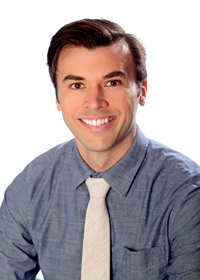
A Department of Energy National Nuclear Security Administration Stewardship Science Graduate Fellowship is part of a team that, for the first time, manipulated photons by changing the orientations of atom-thick layers of materials.
The team, including alumnus Nathan Finney (2015-2019), rotated a two-dimensional layer of hexagonal boron nitride atop a second layer of the material, efficiently modulating and enhancing optical second harmonic generation, in which two incoming photons are combined to produce a single outgoing photon with twice the energy.
The research was published March 3 in the journal Science Advances. Authors include Finney and others from Columbia University, plus scientists from Germany’s Max Planck Institute for the Structure and Dynamics of Matter, Japan’s National Institute for Materials Science and the Simons Foundation Flatiron Institute.
Materials engineers are studying twistronics: how rotating a two-dimensional sheet of material relative to a second sheet changes the entire atomic system’s electronic properties. In a release, Columbia’s James Schuck says the team’s work breaks new ground in a field they call twistoptics – manipulating light by controlling materials in a similar way.

The project targets nonlinear optics, the study of how light interacts with matter. Using the minuscule tip of an atomic-force microscope, they rotated the top boron nitride layer, changing how atoms in each sheet are oriented to each other. That let them precisely tune and enhance optical second harmonic generation.
The techniques could be used for on-chip transducers that couple tiny movements to sensitive optical signals by turning mechanical motion into light. “This is critical for many sensors and devices such as atomic force microscopes,” the release said.
Image caption: Two slabs of boron nitride crystals are dynamically twisted with respect to each other. At certain angles, the incoming laser light (orange beam) can be efficiently converted to higher energy light (pink beam), as a result of micromechanical symmetry breaking. Credit: Columbia University.